Research
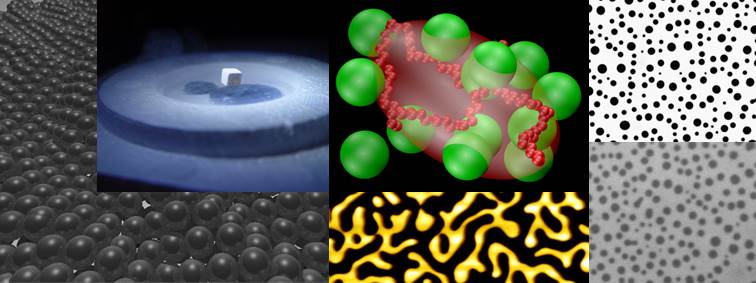
The physics department is a research intensive department with a strong focus on Experimental and Theoretical Soft Condensed Matter Physics and Physics Education Research. Faculty interests also touch on condensed matter and nano-material systems. Soft Matter topics of interest include liquids, polymers, membranes, colloids, grains, and gels. Many of these systems play a central role in industrial applications, or serve as models for biological systems and biomaterials. Our methods to investigate these systems range from phenomenological theoretical modeling and elaborate computer simulations to state of the art experimental techniques such as Atomic Force Microscopy, Confocal Microscopy, Spectroscopy and related laser systems. Some selected topics:
Single-Molecule Electronics and Science
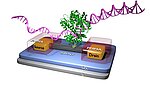
Nanoscale electronic devices like field-effect transistors have long promised to provide sensitive, label-free detection of biomolecules. In particular, single-walled carbon nanotubes have the requisite sensitivity to detect single molecule events, and have sufficient bandwidth to directly monitor single molecule dynamics in real time. With this tool, we investigate the unknown molecular mechanism and complex kinetics of protein activities at a single molecule level.
Involved Faculty: Dr. Yongki Choi
Polymeric Materials
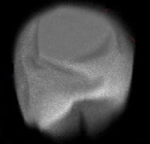
Polymers are one of the most widely used materials in the modern world, yet there are still major challenges in understanding their basic physical behaviour. Our group examines all aspects of these fascinating systems - from molecular details (how do 'tangles' in long chain molecules contribute to their strength?) to macroscopic applications (can thin polymer films form useful origami based mechanical structures? Can large scale complexity in a crumpled ball be related to microscopic features? Can polymers be used to engineer smart drug delivery systems?). We follow these systems with modern tools like atomic force microscopy and confocal microscopy, techniques that are heavily used by the industrial and medical world.
Involved Faculty: Dr. Andrew B. Croll
Fluids
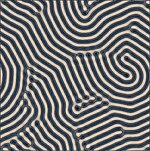
Mixed fluids often exhibit a tendency to phase separate. The structural patterns that are formed upon phase separation evolve in time and space. They depend on dimensionality of the system and on the way the transition is induced. For example, a non-uniform temperature quench can lead to a surprisingly rich dynamic behavior which we study using different analytic and computational methods such as Lattice-Boltzmann simulations.
Involved Faculty:Dr. Alexander Wagner
Physics Education Research
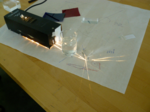
We conduct discipline-based research in Physics Education. The objective is to examine student understanding, and identify and analyze conceptual and reasoning difficulties that students encounter in studying physics. The next step is to design instructional strategies that target specific student difficulties identified by the research, and to assess the effectiveness of these strategies. Therefore, research, curriculum development, and instruction are all integral parts of our investigation.
Involved Faculty: Dr. Mila Kryjevskaia, Dr. Warren Christensen, Dr. John Buncher
Colloids and Polymers
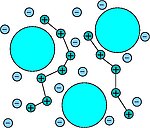
Charged colloids and polymers are widespread in daily life, in nature and technical applications, including paints, cosmetics, emulsions, foods, and sprays. These systems contain macromolecules that are often highly charged so that electrostatic interactions determine the properties and stability of the material. We study charged colloidal systems and polyelectrolytes using analytical theoretical methods and computer simulations.
Involved faculty:Dr. Alan Denton
Membranes
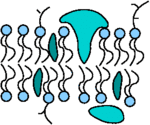
Lipid membranes surround all living cells, forming a barrier that ensures integrity and function. We are interested in understanding the physical properties of membranes and relating them to biological functions. Among the interesting questions are how different lipids influence the lateral organization of a lipid bilayer and what the role of membrane-associated proteins is. Some of our work also addresses bending of lipid membranes and electrostatic interactions between the lipid bilayer and adsorbed macroions.
Involved Faculty: Dr. Sylvio May
Electronic structure of nanoparticles from first principles
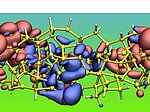
The ability to control properties of nanomaterials via size, shape, composition, surface structure, and self-assembly has opened new degrees of freedom inaccessible in the conventional device design. At the same time, computational studies of nanostructures have become an attractive alternative to the actual experiments, since the ability to explore the vast set of all possible configurations experimentally is limited. In recent years, the combination of advances in the ab initio electronic structure techniques, such as Density Functional Theory, and new computational capabilities has made it possible to perform accurate calculations for the atomistic models of nanoparticles. The results of these studies often serve as a unique source of insight into the system’s properties. Properties of photoexcited semiconductor nanoparticles, such as quantum dots, nanowires, nanofilms, carbon nanotubes, require a description of electrons, photons, and atomic vibrations (phonons), all of which are interacting quantum mechanical particles. Methods of modern quantum field theory, which have been mostly used in theoretical nuclear and particle physics, are combined with the advanced computational electronic structure capabilities to calculate system properties.
Involved Faculty: Dr. Andrei Kryjevski